Introduction:
Microscopic imaging has been a cornerstone of scientific research, providing invaluable insights into the intricate world of biological specimens. However, the resolution limitations of traditional light microscopy have long been a barrier to fully visualizing subcellular structures and activities in detail. In recent years, super-resolution microscopy techniques have emerged as groundbreaking tools that push the boundaries of optical microscopy, allowing researchers to overcome these limitations and reveal the hidden nanoscale intricacies within cells.
Among the array of super-resolution techniques, single-molecule localization microscopy (SMLM) has distinguished itself as a transformative method, revolutionizing the field of cellular imaging. Unlike conventional microscopy, SMLM enables researchers to bypass the diffraction limit of light and capture high-resolution images with exceptional precision. By localizing individual fluorescent molecules with nanometer accuracy, SMLM delivers unprecedented details of cellular structures, opening a new realm of possibilities for understanding cellular dynamics and nanostructures.
In this article, we delve into the multifaceted benefits of SMLM, exploring its working principles and cutting-edge applications that have revolutionized cellular biology. From elucidating the organization of subcellular components to unraveling the complex interactions between molecules, SMLM has emerged as a powerful tool that transforms our understanding of cellular and molecular processes. As we embark on this journey, we will uncover the potential of SMLM in driving breakthroughs in biomedical research and gaining deeper insights into the intricate world that exists at the nanoscale level within living cells.
The Working Principles of Single-Molecule Localization Microscopy:
Traditional light microscopy has long been a reliable tool for visualizing biological samples. However, the diffraction limit of light has imposed a fundamental barrier on the achievable resolution, limiting the ability to observe subcellular structures and nanoscale events in detail. This limitation arises from the wave nature of light, causing light waves to spread out as they pass through the specimen, leading to blurred images with limited resolution.
In recent years, super-resolution microscopy techniques have emerged as transformative solutions to overcome the diffraction limit, pushing the boundaries of what is possible in cellular imaging. Single-molecule localization microscopy (SMLM) is one such technique that has revolutionized the field by enabling researchers to capture images with unprecedented resolution.
The key principle of SMLM is its ability to stochastically activate and deactivate individual fluorescent molecules within the sample. Unlike traditional microscopy, where all fluorescent molecules are excited and emit light simultaneously, SMLM employs a clever approach to image molecules in a sequential manner. By introducing a “blinking” effect, only a small subset of fluorescent molecules is activated and emitting fluorescence at any given time. This stochastic activation is achieved by carefully controlling the excitation and deactivation of fluorescent probes or labels, often through a combination of light intensity and chemical conditions.
Through this clever strategy, SMLM effectively isolates the positions of individual fluorescent molecules, enabling researchers to precisely determine their coordinates in the sample. Sophisticated fitting algorithms are then employed to calculate the precise localization of these molecules based on their emission patterns, achieving localization accuracy at the nanometer scale. As a result, SMLM provides high-resolution positional data for individual molecules, capturing the nanoscale details of the cellular environment that were previously hidden from conventional microscopy.
The collected positional data points are further processed to reconstruct super-resolution images. Unlike traditional microscopy images, which represent the averaged fluorescence emitted by all molecules, SMLM generates images that reveal the precise positions of individual molecules with exceptional spatial resolution. By superimposing the precise localizations of numerous molecules, the final super-resolution image emerges, uncovering the fine structures and nanoscale organization within the specimen.
The benefits of SMLM extend far beyond its ability to break the diffraction barrier. This powerful technique has found diverse applications in cellular biology, neuroscience, and other fields of research. In cellular biology, SMLM has been instrumental in revealing the organization of organelles, the arrangement of membrane proteins, and the spatial distribution of cellular components. In neuroscience, SMLM has shed light on the intricate architecture of synapses and neural networks, advancing our understanding of neuronal function.
Applications in Various Research Fields:
SMLM has indeed revolutionized various scientific disciplines, opening up new frontiers of exploration and discovery. In the field of cell biology, the enhanced resolution offered by SMLM has been instrumental in revealing intricate subcellular structures that were previously inaccessible using conventional microscopy techniques. Researchers can now visualize and study cellular organelles, membrane structures, and cytoskeletal elements with unprecedented detail. This newfound ability to observe cellular components at the nanoscale has significantly advanced our understanding of fundamental cellular processes, including cell division, membrane dynamics, and intracellular signaling pathways. The knowledge gained from SMLM studies contributes to disease modeling and the development of innovative treatments for various medical conditions.
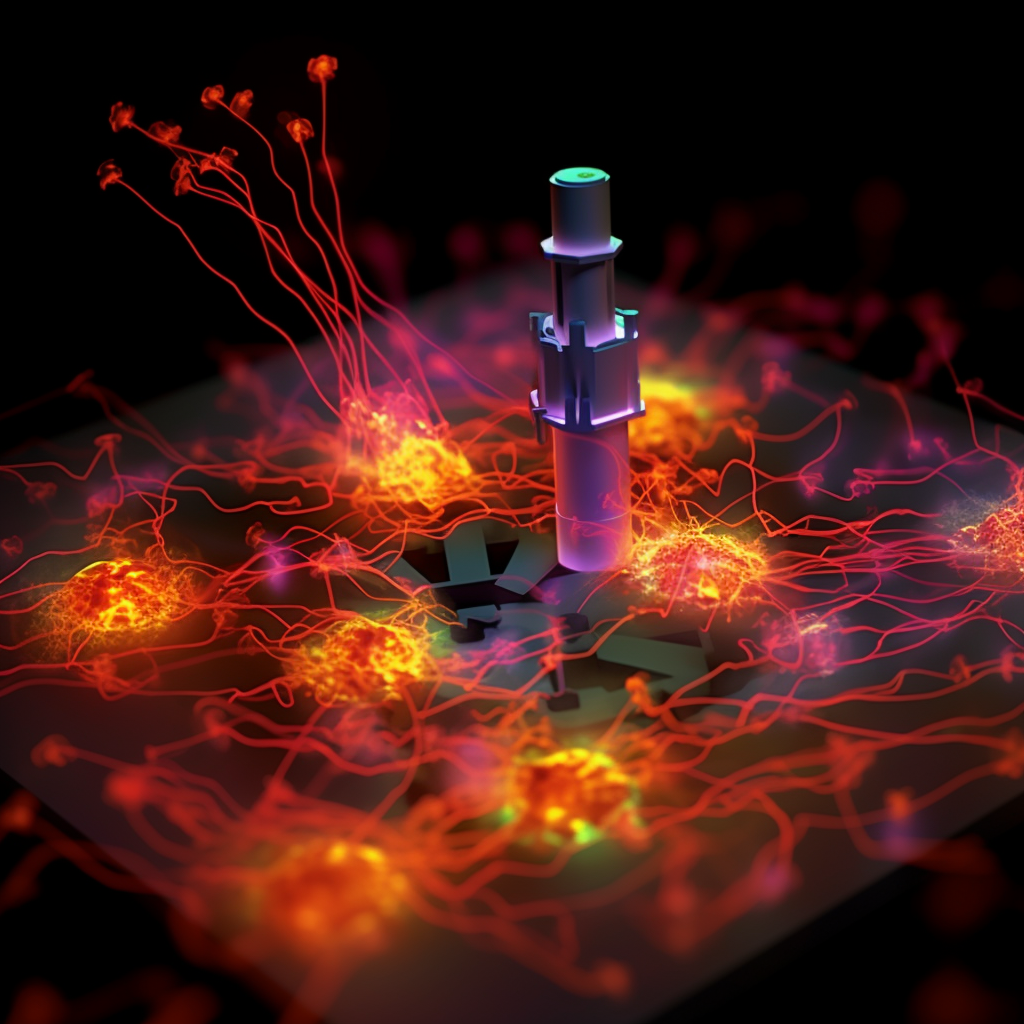
SMLM has also emerged as a powerful tool for investigating the genetic and epigenetic landscape of cells. It has enabled researchers to probe the spatial organization of DNA, RNA, and proteins within the nucleus. By studying macromolecular complexes, chromatin structure, chromosomal substructures, and genome organization at the nanoscale, SMLM has provided critical insights into gene regulation and genome function. This deep understanding of the spatial arrangement of genetic information within a single nucleus has implications for fields such as developmental biology, cancer research, and regenerative medicine.
In the domain of spatial biology research, SMLM has proven invaluable for studying small entities such as viruses and extracellular vesicles (EVs). These entities are often below the resolution limit of other microscopes, making them challenging to observe and characterize accurately. However, with the high-resolution imaging capabilities of SMLM, researchers can now investigate the interactions, intracellular movement, and structures of viruses and EVs in detail. This knowledge is crucial for advancing our understanding of their biology, replication mechanisms, and potential therapeutic applications. In the context of infectious diseases, SMLM has been instrumental in studying viral-host interactions and the mechanisms of viral entry into cells, leading to the development of targeted antiviral strategies.
The field of neuroscience has also greatly benefited from the implementation of SMLM. By enabling detailed studies of synapses, SMLM has provided critical insights into the complex workings of the brain. Synapses are the essential connections between neurons and play a fundamental role in neural communication and information processing. SMLM has allowed researchers to visualize the nanoscale organization of synaptic proteins and the spatial distribution of neurotransmitter receptors. These findings have significant implications for understanding synaptic plasticity, neuronal connectivity, and synaptic dysfunction in neurological and psychiatric disorders.
Moreover, SMLM has facilitated the exploration of super-resolution imaging in living organisms. By combining SMLM with advanced imaging techniques such as fluorescence microscopy and time-lapse imaging, researchers can now study dynamic cellular processes and molecular interactions in real-time with unprecedented resolution. This capability has opened up new avenues for studying cellular responses to stimuli, dynamic protein localization, and cell signaling events, providing deeper insights into the mechanisms that underlie cellular behavior and function.
Bruker’s Vutara VXL is an example of a dedicated super-resolution microscope that offers superior resolution performance and stability. It utilizes a single objective and controls temperature gradients to ensure reliable imaging. Bruker’s Vutara SRX software, coupled with the Vutara VXL, empowers researchers to extract both visual and quantitative information from biological samples. The software simplifies experimentation, guides users through the workflow, and provides comprehensive analysis tools, enhancing researchers’ ability to derive meaningful insights from their SMLM data.
The Future of Super-Resolution Microscopy:
The future of super-resolution microscopy holds immense potential for further breakthroughs in various life science fields. As research progresses, there is a growing realization that combining different imaging methods through correlative microscopy yields even greater benefits. Correlative microscopy, particularly the integration of electron microscopy (EM) with SMLM, enables targeted labeling and ultra-high-resolution imaging. This powerful combination allows researchers to collect rich and in-depth data that goes beyond the capabilities of any single technique, opening new avenues for scientific exploration.
One of the key advantages of correlative microscopy is its ability to provide complementary information about cellular structures and processes. While SMLM offers exceptional resolution and the ability to visualize specific molecules or subcellular components, electron microscopy excels in capturing ultra-high-resolution images of cellular ultrastructure. By combining the strengths of both techniques, researchers can precisely localize labeled molecules within the context of their cellular environment, providing a comprehensive view of cellular organization and dynamics.
Moreover, correlative microscopy is not limited to the integration of SMLM and electron microscopy. It can also incorporate other imaging modalities, such as fluorescence microscopy, confocal microscopy, and light-sheet microscopy, to extract additional information from biological samples. This multi-modal approach allows researchers to obtain a more comprehensive understanding of cellular processes and interactions, paving the way for new discoveries in fields such as cell biology, neuroscience, developmental biology, and cancer research.
Advancements in super-resolution microscopy will continue to deepen our understanding of complex biological processes. As researchers examine biological samples at even higher resolutions, they gain insights into molecular interactions, cellular signaling pathways, and organelle dynamics that were previously hidden from view. This detailed understanding of cellular mechanisms has profound implications for the development of new drugs, treatment strategies, and interventions targeting specific disease processes.
In the realm of medicine, the ability to visualize and study subcellular structures deep within samples through SMLM holds great promise for improving patient health outcomes. Super-resolution microscopy is increasingly being used in medical research and diagnostics, enabling researchers and clinicians to better understand the molecular basis of diseases and develop targeted therapies. By elucidating the underlying molecular mechanisms of diseases, super-resolution microscopy can aid in the identification of potential biomarkers, drug targets, and personalized treatment approaches.
As the field of super-resolution microscopy continues to advance, there is also a focus on making these techniques more accessible and user-friendly. Improvements in microscope hardware, software, and data analysis tools aim to simplify the process of acquiring and interpreting high-resolution images. This democratization of super-resolution microscopy will empower more researchers to leverage its capabilities and contribute to a deeper understanding of biology and medicine.
Conclusion:
In conclusion, single-molecule localization microscopy (SMLM) has ushered in a new era of biological research, providing scientists with the unprecedented ability to explore the nanoscale world of living organisms. By overcoming the limitations of traditional microscopy, SMLM has enabled the visualization of subcellular structures and activities with exceptional resolution, unraveling the intricacies of cellular dynamics and nanostructures. Its applications extend across a wide range of research fields, from cell biology to neuroscience, genomics to virology, and spatial biology research.
To harness the full potential of SMLM, researchers can opt for dedicated super-resolution microscopes such as Bruker’s Vutara VXL, which offer superior resolution performance and stability. The availability of cutting-edge microscopy tools empowers scientists to delve deeper into the mysteries of life at the molecular level, contributing to the advancement of medical knowledge and the development of innovative treatments.
Looking ahead, the future of super-resolution microscopy holds tremendous promise. The integration of SMLM with other imaging modalities, such as correlative microscopy, will provide a comprehensive view of complex biological processes, offering a deeper understanding of cellular function and interactions. Ongoing advancements in super-resolution techniques and instrumentation will further enhance the capabilities of SMLM, fueling groundbreaking discoveries in various research domains.
As the boundaries of SMLM continue to expand, its impact on healthcare outcomes is expected to be substantial. By uncovering the finer details of cellular processes and disease mechanisms, super-resolution microscopy will pave the way for targeted therapies, precision medicine, and personalized treatments.
SMLM has transformed biological research, unlocking the potential to observe and comprehend the intricate workings of life at the nanoscale. With dedicated super-resolution microscopes and ongoing advancements in the field, the future of super-resolution microscopy is bright, promising to revolutionize our understanding of complex biological systems and drive improvements in human health. Embracing this powerful imaging technology will undoubtedly lead to groundbreaking discoveries and innovations that will shape the future of medicine and biology.
If you need more information on this article click on the following link. This link will provide more extensive information and elaborate on the topic.
Genomic.News is your ultimate source for curated news, articles, and updates on the fascinating world of genomics. Our platform brings together the latest scientific breakthroughs, research advancements, and industry developments in one centralized hub. Stay informed about the cutting-edge discoveries, transformative technologies, and ethical considerations driving the field of genomics. Explore the intersection of genetics and healthcare, agriculture, biotechnology, and beyond.